08.12.2015 - CTA Prototype Telescope Achieves "First Light"
|
|
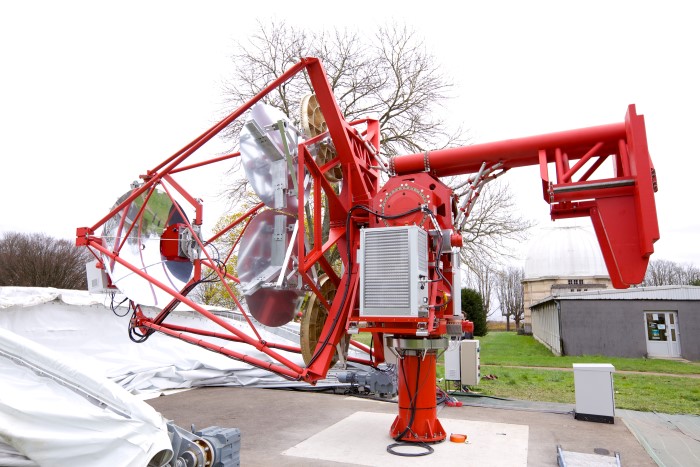
|
|
Left: GCT camera; right: GCT prototype on site the Observatoire de Paris, Meudon, France.
(credit: Akira Okumura)
|
On 2015, November 26, a prototype telescope proposed for the Cherenkov Telescope Array, the Gamma-ray Cherenkov Telescope (GCT), recorded CTA’s first ever Cherenkov light while undergoing testing at l’Observatoire de Paris in Meudon, France. The GCT is proposed as one of CTA’s small size telescopes (SSTs), covering the high end of the CTA energy range, between about 1 and 300 TeV (tera-electronvolts). Another SST prototype, the ASTRI telescope, captured the first optical image in May 2015 with its diagnostic camera.
In the two weeks leading up to the GCT prototype inauguration event on December 1, the GCT team battled poor weather to install and begin testing the GCT camera, left picture on top. On the evening of November 26, they turned the telescope away from a nearly full moon and the bright lights of Paris towards a clear patch of sky. After 20 seconds, a single event triggered the camera, then another – in just over 300 seconds 12 events were captured. These triggers could have been caused by fluctuations in the bright night sky, but it was instantly clear that they were, in fact, what the team was looking for – images of air showers created in the atmosphere by cosmic rays.
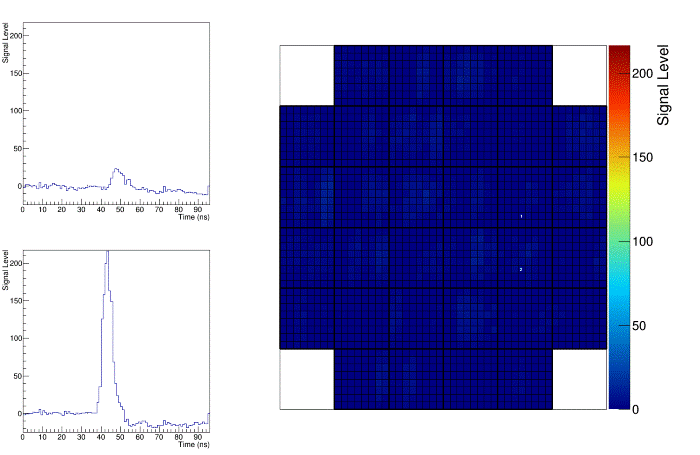
Image of an event captured by the GCT
(credit: CTA Collaboration)
The image shown below is one of the events captured by the team. It shows the maximum amount of light captured in each of the camera’s 2048 pixels over 100 frames. CTA astronomers will use images like this to determine the incoming direction and energy of the particle that created the air shower.
"With the tough weather conditions, we only had about an hour-long window to gather as much data as we could," said GCT Camera Coordinator Dr. Richard White. "We look forward to clearer, darker skies so we can test the camera’s performance in more ideal conditions."
"This is a major milestone for the GCT and we hope for CTA." said GCT Spokesperson Prof. Tim Greenshaw. "Our design for the CTA telescopes that will detect the highest energy light hitting the earth’s atmosphere from space has been proven to work; we are one step closer to developing a deeper understanding of where and how that light is produced."
Hélène Sol, Research Director at Centre National de la Recherche Scientifique (CNRS) and GCT Deputy Spokesperson added: "I would like to congratulate all the GCT team who have made this possible, especially the group who worked day and night over the last couple of weeks to get these pictures."
In order to detect the short flashes of light produced by cosmic rays and gamma rays as they hit the earth’s atmosphere, the telescope's camera has to be about a million times faster than a DSLR camera. To do this, it uses high-speed digitisation and triggering technology capable of recording images at a rate of one billion frames per second and sensitive enough to resolve single photons.
These first pictures are just the beginning for the GCT. The prototype telescope and camera will undergo rigorous testing over the next year, then the team intends to build 35 cameras and telescopes for the CTA Observatory based on the results of the testing process.
"We’re extremely pleased with the progress and performance of the GCT prototype and all of the CTA prototypes," said CTA Project Manager Christopher Townsley. "We look forward to seeing the results of further testing as we near the construction phase of the project."
CTA
CTA is a global initiative to build the world’s largest and most sensitive high-energy gamma-ray
observatory. Over 1,000 scientists and engineers from 32 countries and over 170
research institutes participate in the CTA project. CTA will serve as an open observatory to a
wide physics and astrophysics community and provide a deep insight into the non-thermal,
turbulent, high-energy universe. The CTA observatory will detect high-energy radiation with
unprecedented accuracy and approximately 10 times the sensitivity of current instruments,
providing novel insights into some of the most extreme and violent events in the universe.
At least three telescope types are required to cover the full CTA energy range. The sensitivity
in the core energy range between 100 GeV and 10 TeV will be dominated by up to 40
Medium-Size Telescopes (MSTs) distributed over both array sites in the northern and
southern hemispheres. Four Large-Size Telescopes (LSTs) and around 70 SSTs will be
essential to extend the energy range below 100 GeV and above a few TeV. The GCT adds to
the current complement of CTA prototypes located around the world: the SST-1M (Krakow,
Poland), SST-2M ASTRI (Serra la Nave, Italy), MST (Zeuthen, Germany) and the LST (La
Palma, Spain).
|
Press release: CTA
08.12.2015 - The construction of KM3NeT, the next generation neutrino telescope, has begun
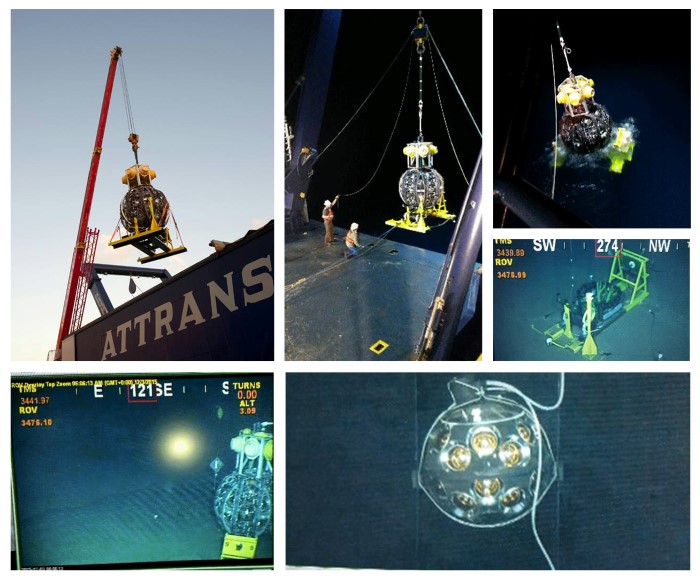
The neutrino detection string wound around the spherical deployment frame prior to
deployment on board the boat to touch down of the deployment frame
on the sea floor
(underwater pictures: Display screens in control room).
(credit: KM3Net Collaboration)
In the early morning of 2015 December 3, scientists and engineers started the installation of
KM3NeT, which once completed, will be largest detector of neutrinos in the Northern
Hemisphere. Located in the depths of the Mediterranean Sea, the infrastructure will be used
to study the fundamental properties of neutrinos and map the high-energy cosmic neutrinos
emanating from extreme cataclysmic events in the Universe.
On board the Ambrosius Tide deployment boat, the first string – wound, like a ball of wool, around a spherical frame – arrived at the location of the KM3NeT-Italy site south of Sicily. It was anchored to the seabed at a depth of 3,500 m and connected to a junction box, already present on the seafloor, using a remotely operated submersible controlled from the boat. The junction box is connected by a 100 km cable to the shore station located in Portopalo di Capo Passero in the south of Sicily.
Marco Circella, the technical coordinator of KM3NeT explains, "The large depth of sea water shields the telescope from particles created by cosmic rays in the atmosphere above the telescope. Constructing such a large infrastructure at these depths is a tremendous technical challenge. Making the underwater connections requires custom-designed electrical and fibre optic connectors. The crew of the Ambrosius Tide are experts in performing such delicate submarine operations."
After verification of the quality of the power and fibre optic connections to the shore station, the go ahead was given to trigger the unfurling of the string to its full 700 m height. During this process, the deployment frame is released from its anchor and floats towards the surface while slowly rotating. In doing so, the string unwinds from the spherical frame, eventually leaving behind a vertical string. The string was then powered on from the shore station and the first data from the sensor modules started streaming to shore.
The successful acquisition of data from the abyss with the novel technology developed by the KM3NeT Collaboration is a major milestone for the project. It represents the culmination of more than ten years of research and development by the many research institutes comprising the international Collaboration.
Maarten de Jong, spokesperson and director of KM3NeT, said: “This important step in the verification of the design and the technology will allow the KM3NeT Collaboration to proceed with confidence toward the mass production of detection strings and their installation at thesites in the Mediterranean Sea off-shore from Italy and France. A new era in neutrino astronomy has begun”
KM3Net
Neutrinos are the most elusive of elementary particles and their detection requires the instrumentation of enormous volumes: The KM3NeT neutrino telescope will occupy more than a cubic kilometre of seawater. It comprises a network of several hundred vertical detection strings, anchored to the seabed and kept taut by a submerged buoy. Each string hosts 18 light sensor modules equally spaced along its length. In the darkness of the abyss, the sensor modules register the faint flashes of Cherenkov light that signal the interaction of neutrinos with the seawater surrounding the telescope.
The international KM3Net Collaboration consists of 35 research groups from France, Germany, Greece, Italy, Morocco, The Netherlands, Poland and Spain.
|
Press release: KM3Net
02.12.2015 - A search for cosmic-ray sources with IceCube, the Pierre Auger Observatory, and the Telescope Array
High-energy neutrinos are thought to be excellent cosmic messengers when exploring the extreme universe: they don’t bend in magnetic fields as cosmic rays (CRs) do and they are not absorbed by the radiation background as gamma rays are. However, it turns out that the deviation of some CRs, namely protons, is expected to be only a few degrees at energies above 50 EeV. This opens the possibility for investigating common origins of high-energy neutrinos and CRs.
In a new study by the IceCube, Pierre Auger, and Telescope Array Collaborations, scientists have looked for correlations between the highest energy neutrino candidates in IceCube and the highest energy CRs in these two cosmic-ray observatories. The results, submitted today to the Journal of Cosmology and Astroparticle Physics, have not found any correlation at discovery level. However, potentially interesting results have been found and will continue to be studied in future joint analyses.
|
|
|
|
Maps in Equatorial and Galactic coordinates showing the arrival directions of the IceCube cascades (black dots) and tracks (diamonds), as well as those of the UHECRs detected by the Pierre Auger Observatory (magenta stars) and Telescope Array (orange stars). The circles around the showers indicate angular errors. The black diamonds are the HESE tracks while the blue diamonds stand for the tracks from the through-going muon sample. The blue curve indicates the Super-Galactic plane. Image: IceCube, Pierre Auger and Telescope Array Collaborations.
(credit: IceCube, Pierre Auger, TA collaborations)
|
|
The IceCube astrophysical neutrino flux is consistent with an isotropic distribution, which suggests that most neutrinos have an extragalactic origin. The intensity of this flux is also found to be close to the so-called Waxman-Bahcall flux, which is the rate assuming that ultra-high-energy CRs (UHECRs) are mainly protons and have a power-law
spectrum. In this scenario, primary cosmic rays collide to a significant extent with photons and neutrons within the source environment, resulting in mainly protons escaping from these sources.
The UHECRs detected by the Pierre Auger Observatory (Auger) and the Telescope Array (TA) that were used in this study have energies above 50 EeV, since at the highest energies cosmic rays are deflected the least. UHECRs produce neutrinos that carry only 3-5% of the original proton energy, i.e., neutrinos that would have energies of at least several hundred PeVs for the CR sample of this work. However, we expect that the sources of these UHECRs will also produce lower energy CRs, which would then produce neutrinos in the energy range—30 TeV to 2 PeV—observed in IceCube. And this is the idea behind this search: to look for a statistical excess of neutrinos in IceCube from the direction of cosmic rays in the Auger and TA and, thus, their sources.
Not a simple search, but definitely worth trying to study since searches for the most obvious potential CR sources using IceCube neutrinos have not been successful so far. The major challenges of this search are: i) CRs do not precisely point to their sources, and our knowledge of the deviations produced by the galactic magnetic fields is limited; ii) cascade neutrino events—mainly produced by electron and tau neutrinos—in IceCube are characterized by large angular uncertainties; and iii) IceCube neutrino candidates include background muon events due to the interaction of CRs with the Earth’s atmosphere.
Researchers have used three different analyses to tackle these challenges. They first searched for cross-correlations between the number of CR-neutrino pairs at different angular windows and compared them to expectations for the null hypothesis of an isotropic UHECR flux. Then, they used a stacking likelihood analysis that looked for the combined contribution from different sources. These two searches used both cascade and track neutrino events from the astrophysical neutrino fluxes measured in IceCube (HESE and high-energy throughgoing muons). IceCube track neutrino signatures are produced by charged-current interactions of muon neutrinos and have an angular uncertainty of less than one degree. Finally, they performed a third study, a stacking search using the neutrino sample used for the four-year point-source search in IceCube, which includes track neutrino candidates only.
The results obtained are all below 3.3 sigma. There is a potentially interesting finding in the analyses performed with the set of high-energy cascades. When compared to an isotropic flux of neutrinos (fixing the positions of the cosmic rays) to consider the effect of anisotropies in the arrival directions of cosmic rays, the significance is 2.4 sigma for the cross-correlation analysis. These results were obtained with relatively few events and the collaborations will update the analyses in the future with additional statistics to follow their evolution.
Press release: IceCube, Pierre Auger Observatory
Publication: Search for correlations between the arrival directions of IceCube neutrino events and ultrahigh-energy cosmic rays detected by the Pierre Auger Observatory and the Telescope Array, IceCube, Pierre Auger and Telescope Array Collaborations: M.G.Aartsen et al., submitted to Journal of Cosmology and Astroparticle Physics (arXiv:1511.09408)
16.11.2015 - AugerPrime Symposium

Celebrating 15 years of achievements and
signature ceremony of a new International Agreement for the next 10 years
The Pierre Auger Observatory is the world’s leading science project for the exploration of cosmic rays. More than 500 scientists from 16 countries have been working together since 1998 in the Province of Mendoza, Argentina, to elucidate the origin and properties of the most energetic particles in the Universe, coming to us from the far reaches of the cosmos. The Pierre Auger Observatory measures gigantic showers of relativistic particles that are the result of collisions between the very rare, highest-energy cosmic rays and atomic nuclei of the atmosphere. Properties of such air showers are used to infer the energy, direction, and mass of the cosmic particles.
Results from the Pierre Auger Observatory have brought new fundamental insights into the origin and nature of highest-energy cosmic rays. One of the most exciting results is the experimental proof that at the highest energies (7 orders of magnitude above what can be achieved at CERN’s Large Hadron Collider) the cosmic-ray flux decreases much faster than at low energies. Data indicate that this flux suppression marks the limiting energy of the most powerful cosmic particle accelerators. An even more detailed measurement of the nature of cosmic particles at the highest energies is crucial to understand the mechanisms responsible for this decrease, and to identify the astrophysical sites violent enough to accelerate particles to such tremendous energies.
The AugerPrime upgrade to the Observatory enhances the 1660 existing surface detectors (water tanks sensitive to Cherenkov light generated by the shower products) with new scintillation detectors, so that electromagnetic and muonic shower particles can be separated more efficiently. This in turn, together with smaller area of buried muon detectors, improves the determination of the mass of the primary cosmic rays, otherwise not directly measurable. Faster and more powerful electronics also facilitates the readout of the new detector components and enhances the overall performance of the Observatory elements.
A symposium, held on November 15-16, 2015 gathers collaborators and science funding agency representatives for the signing of a new international agreement for continued operation of the Pierre Auger Observatory until 2025. This will provide the basis for doubling the present statistics with the upgraded Observatory, and for solving the long-standing puzzle of the origin of the most energetic particles in the Universe.
|
 |
 |
|
A prototype station of AugerPrime in the Argentinian Pampa: The Water Cherenkov Detector containing 12000 liters of water (bottom) is augmented by a 4 square meter scintillation detector (top) to enhance the particle identification capabilities. |
Headquarter of the Pierre Auger Observatory in Malargüe, Province of Mendoza, in Argentina. |
|
 |
 |
|
Group picture of the participants of the AugerPrime Symposium. |
AugerPrime Symposium. |
|
 |
|
|
AugerPrime Symposium. From left to right: Prof. Karl Heinz Kampert, spokesperon of the Pierre Auger Observatory, Bergische Universität Wuppertal, Germany; Alberto Lamagna, Gerente de Área de Investigación y Aplicaciones No Nucleares de la Comisión Nacional de Energía Atómica (CNEA), Argentina; Dr. Lino Barañao, Ministro de Ciencia, Tecnología e Innovación Productiva; Juan Antonio Agulles, Intendente del Departamento de Malargüe, Argentina; Prof. Fernando Ferroni, Presidente of the Instituto Nazionale di Fisica Nucleare, Italy; Ing. Julio Cobos, Senador Nacional Electo por la Provincia de Mendoza, Argentina; Ing. Rolando Baldasso, Ministro de Infraestructura de la Provincia de Mendoza. |
Signature ceremony. Were present from left to right: Prof. Mario-Pimenta, LIP, Portugal; Dr. Lino Barañao, Ministro de Ciencia, Tecnología e Innovación Productiva; Dr. Carola Dobrigkeit, UNICAMP, Brazil; Prof. Fernando Ferroni, INFN, Italy; Prof. Johannes Bluemer, KIT, Karlsruhe, Germany; Jan Ridky, ELI, Czech Republik; Alberto Lamagna, CNEA, Argentina; Dr. Reynald Pain, CNRS - IN2P3, Paris, France; Prof. Stan Bentvelsen, Nikhef, Netherlands; Ing. Rolando Baldasso, Ministro de Infraestructura de la Provincia de Mendoza. |
Material: Pictures above (high-res, web-HD), individual pictures of signatory (high-res, web-HD).
Press release: Pierre Auger Observatory
11.11.2015 - XENON1T inauguration at Gran Sasso
.JPG)
XENON1T inauguration
(credit: INFN-LNGS)
On November 11, an international collaboration of scientists inaugurated the new XENON1T instrument designed to search for dark matter with unprecedented sensitivity, at the Gran Sasso Underground Laboratory of INFN in Italy.
The event took place at the Gran Sasso National Laboratory of the Italian National Institute for Nuclear Physics (INFN-LNGS), the largest underground laboratory in the world for astroparticle physics.
The inauguration was attended by the XENON scientists along with guests from funding agencies as well as journalists and colleagues. About 80 visitors were able to join the ceremony directly at the experimental site in the 100 m-long, 20 m-wide and 18 m-high hall B of LNGS, which is itself below 1 400m of rock. Here, the new XENON1T instrument is installed inside a 10 m-diameter water tank to shield it from radiation which originates from the environment. Even more guests followed the introductory presentations in the LNGS auditorium, where Elena Aprile, Professor at Columbia University (New York) and founder of the XENON project, illustrated the evolution of the XENON program from the early beginnings with a 3 kg detector 15 years ago to the present-day instrument XENON1T with a total mass of 3 500kg.
Once fully operational, XENON1T will be the most sensitive dark matter experiment in the world. The detector installation has been completed just a few days ago and the first tests of its performance have already been started. The first science results are expected early 2016, as only one week of good data is sufficient to yet again take the lead in the field. The design goal of the experiment will be reached after two years of data taking, as the collaboration explains in a detailed sensitivity study published at the same time as the inauguration. The ultimate goal is the detection of the dark matter particle. Still, even if there are only some hints found after two years of operation, the XENON collaboration will be in an excellent position to move forward, as the next phase of the project, XENONnT, is already being prepared. It will largely use already existing infrastructure, and will increase the sensitivity to dark matter by another order of magnitude
Dark Matter
Dark matter is one of the basic ingredients of the Universe, and efforts to detect it with laboratory-based experiments have been ongoing for decades. However, until today dark matter has been observed only indirectly via its gravitational interactions – the interactions that govern the dynamics of the Cosmos at all length-scales. It is expected that dark matter is made of a new, stable elementary particle which has so far escaped detection. About 100 000 dark matter particles are expected to pass through an area of 1 cm² per second. The fact that these particles have not yet been directly detected puts stringent constraints on their tiny interaction probability with the atoms of ordinary matter. It also implies that more sensitive instruments are required to find the rare signature of the dark matter particle.
|
XENON1T
XENON1T employs the noble gas xenon as the dark matter detection material, which must be made ultra-pure and cooled down to –95°C to make it liquid. The large-mass instrument features an extremely low radioactive background in order to be able to identify the rare events from dark matter interactions. For this reason, the XENON scientists have carefully selected all materials used in the construction of the detector, ensuring that their intrinsic contamination with radioactive isotopes meets the experiment's low-background strict requirements.
The XENON1T detector measures the tiny flashes of light and charge which are generated when a particle interacts with the xenon. The scientists use this information to reconstruct the position of the particle interaction within the detector, as well as the deposited energy and whether the interaction may have been induced by dark matter. The light is observed by 248 sensitive photosensors, which are each capable of detecting even single photons. A vacuum-insulated double-wall cryostat, essentially a gigantic version of a thermos flask, contains the cryogenic xenon and the dark matter detector. The xenon gas is cooled down and purified in the three-story tall XENON building, a fancy installation with a transparent glass facade right next to the water tank, allowing visitors to actually see what the scientists are doing inside. A gigantic stainless-steel sphere equipped with pipes and valves is installed on the ground floor. It can accommodate 7.6 tons of xenon in liquid and gaseous form, more than two times the capacity needed for XENON1T. This will allow the collaboration to swiftly increase the sensitivity of the experiment by using a larger mass detector in the near future.
The international XENON Collaboration, consisting of 21 research groups from the United States, Germany, Italy, Switzerland, Portugal, France, the Netherlands, Israel, Sweden and United Arab Emirates, celebrated the inauguration of their new XENON1T instrument today, which will search for dark matter with unprecedented sensitivity.
|
Press release: INFN
10.11.2015 - Breakthrough Prize in Fundamental Physics für Neutrinophysiker
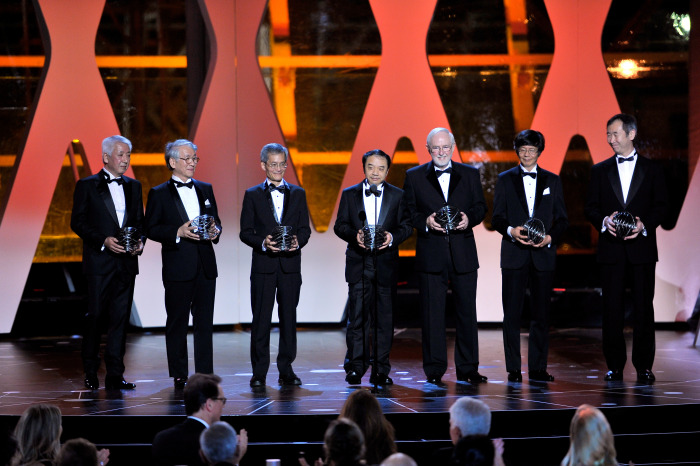
Breakthrough winners onstage during the 2016 Breakthrough Prize Ceremony
on November 8, 2015 in Mountain View, California.
(Photo by Steve Jennings/Getty Images for Breakthrough Prize)
(Copyright Getty Images 2015)
Wissenschaftler von fünf Experimenten erhalten gemeinsam den 2016 Breakthrough Prize in Fundamental Physics. Die Forscher haben grundlegende Arbeiten zur Entdeckung und Erforschung von Neutrino-Oszillationen geleistet und damit wichtige Einblicke in Vorgänge im Universum erhalten, so die Begründung der Jury. Unter den Ausgezeichneten ist auch der Dresdner Physiker Kai Zuber. Er teilt sich den Preis mit insgesamt 1376 Kollegen, die wie er an einem der fünf Großexperimente arbeiten: Daya Bay (China), KamLAND (Japan), K2K / T2K (Japan), Sudbury Neutrino Observatory SNO (Kanada) und Super-Kamiokande (Japan). Der Breakthrough Prize ist mit insgesamt 3 Millionen US-Dollar dotiert und wird von einer Stiftung vergeben, die von dem russischen Unternehmer Juri Milner gegründet wurde.
Schon der Physik-Nobelpreis wurde in diesem Jahr an Neutrino-Physiker vergeben: an Takaaki Kajita und Arthur B. McDonald, die den Super-Kamiokande-Detektor beziehungsweise das SNO Experiment leiten. Mit dem Breakthrough Prize werden nun explizit alle Wissenschaftler ausgezeichnet, die an den Arbeiten beteiligt waren. Dazu gehört auch Kai Zuber, Professor für Kernphysik an der TU Dresden, der seit 15 Jahren bei SNO mitarbeitet.
Neutrinos sind Elementarteilchen, die in drei verschiedenen Arten vorkommen. Diese Arten können sich ineinander umwandeln, was mit dem Begriff Neutrino-Oszillationen beschrieben wird. Dieser Vorgang wurde an den nun ausgezeichneten Experimenten untersucht und nachgewiesen. Außerdem ergaben die Versuche, dass Neutrinos nicht masselos sind – wie lange angenommen wurde. Die Dresdner Kernphysiker um Kai Zuber arbeiten nun am Nachfolgeexperiment von SNO, genannt SNO+. Außerdem sind Zuber und seine Gruppe an weiteren Neutrino-Experimenten wie z.B. GERDA oder COBRA beteiligt. Die Untersuchungen haben unter anderem zum Ziel, die genauen Werte der Neutrinomassen zu bestimmen.
Pressemitteilung: TU Dresden
06.10.2015 - 2015 Nobel Prize in Physics for Astroparticle Physics
The Royal Swedish Academy of Sciences has decided to award the Nobel Prize in Physics for 2015 to Takaaki Kajita (University of Tokyo) and Arthur B. McDonald (Queen’s University) "for the discovery of neutrino oscillations, which shows that neutrinos have mass".
The discovery rewarded with the 2015 Nobel Prize in Physics have yielded crucial insights into the all but hidden world of neutrinos. After photons, neutrinos are presumably the most numerous in the entire cosmos. The Earth is constantly bombarded by them. Many neutrinos are created in reactions between cosmic radiation and the Earth’s atmosphere. Others are produced in nuclear reactions inside the Sun. Thousands of billions of neutrinos are streaming through our bodies each second. Hardly anything can stop them passing; neutrinos are nature’s most elusive known elementary particles.
The possibility of neutrino flavour change, i.e. neutrino oscillations, has been discussed already shortly after neutrinos were first discovered experimentally in 1956. However, it was around the turn of the millennium that two convincing discoveries validated the actual existence of neutrino oscillations.
In 1998, at Neutrino’98, the largest international neutrino conference series, Takaaki Kajita of the Super-Kamiokande Collaboration presented the first evidence that neutrinos from the atmosphere switch between two identities on their way to the Super-Kamiokande detector in Japan. Also miraculous had been the measured flux of electron-type neutrinos from the Sun which appeared to be only about 1/3 of the theoretical expectation.
In addition, in 2001/2002, the Sudbury Neutrino Observatory (SNO) Collaboration, led by Arthur B. McDonald, were able to measure both, the electron-type neutrino flux and the total neutrino flux of all flavours from the Sun. They confirmed a deficit in electron-type neutrinos – which lead to the 2002 Nobel Prize in Physics to Raymond Davis and Masatoshi Koshiba. In 2004 SNO published clear evidence for conversion of electron-type neutrinos from the Sun into muon-or tau-neutrinos by observing the expected neutrino flux from solar fusion reactions.
The discovery that neutrinos can convert from one flavour to another and therefore have non-zero masses is a major milestone for elementary particle physics and astroparticle physics. It represents compelling experimental evidence for the incompleteness of the Standard Model – which requires neutrinos to be massless – as a description of nature.
Today, understanding the nature of the neutrino is of prime importance – not only for elementary particle physics but also for astrophysics and cosmology. The answer to several key questions about the nature of the neutrino may lead to the discovery of new theories beyond the Standard Model. What are the neutrinos’ masses? Why are they so light-weight? Are there more types than the three currently known? Are neutrinos their own antiparticles? Why are they so different from other elementary particles? Neutrino oscillations and the connected issues of the nature of the neutrino, neutrino masses and possible CP violation among leptons are today major research topics in particle and astroparticle physics.
The discovery that is awarded with this year’s Nobel Prize in Physics has yielded crucial insights into the almost entirely hidden world of the neutrino. The prize is received at a time where the investigations are continued and research of neutrino receives an unprecedented attention around the world. Several neutrino experiments are key projects of the Helmholtz Alliance for Astroparticle Physics and pursued by the participating scientists. New discoveries of the neutrino’s closely guarded secrets are expected and will continue to change our understanding of the history, structure and future fate of the universe.
2015 Nobel Prize Laureates
Takaaki Kajita was born in 1959 in Higashimatsuyama, Japan. He earned his PhD in 1986 from University of Tokyo, Japan. He is currently director of the Institute for Cosmic Ray Research and a professor at University of Tokyo in Kashiwa, Japan.
In 2013 Takaaki Kajita was laureate of the Julius Wess Award of KCETA at the Karlsruhe Institute of Technology (KIT).
Arthur B. McDonald was born in 1943 in Sydney, Canada. He earned his PhD in 1969 from California Institute of Technology in Pasadena, California. He is a professor emeritus at Queen’s University in Kingston, Canada.
|
Sudbury Neutrino Observatory
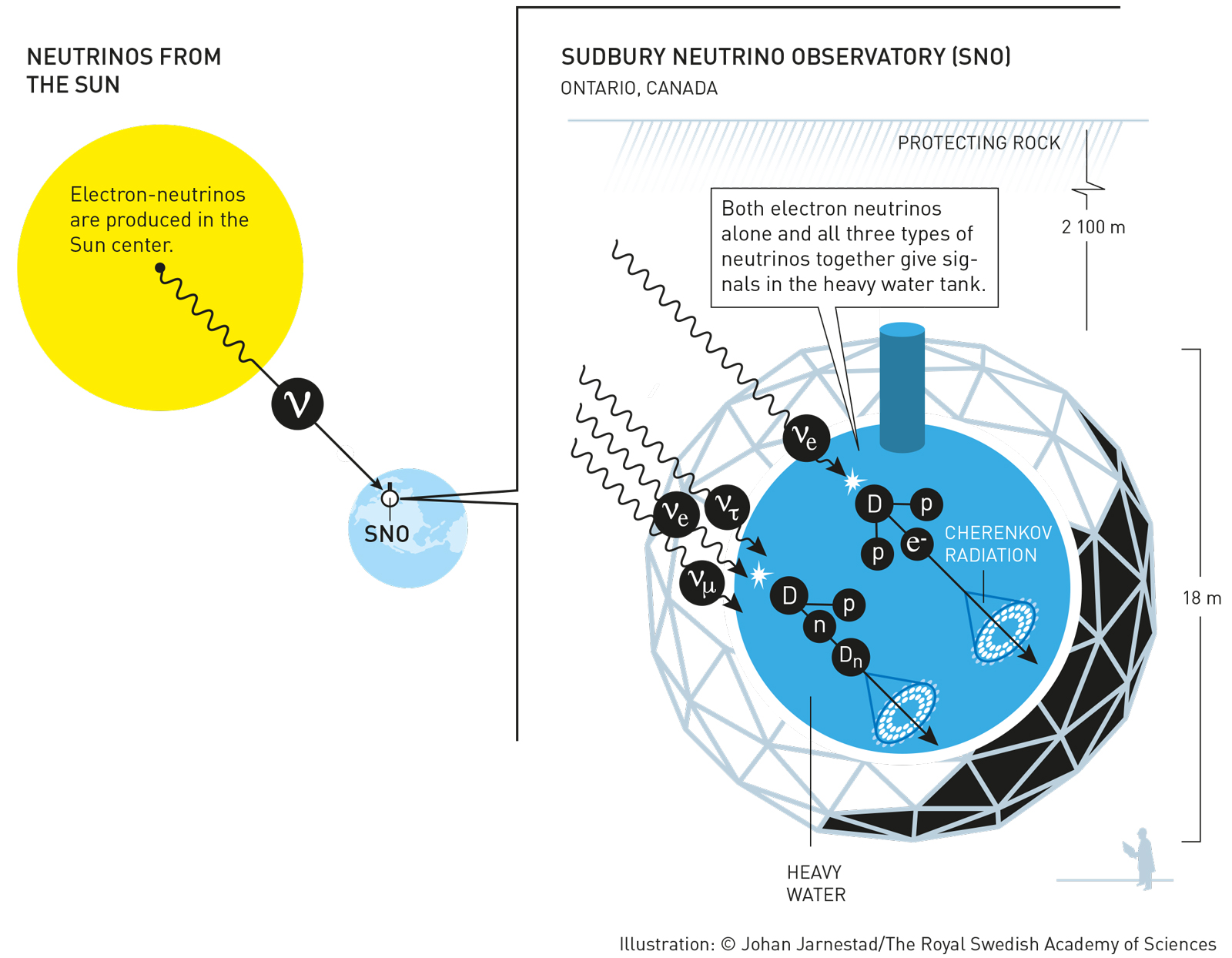
Sudbury Neutrino Observatory detects neutrinos from the Sun.
Sudbury Neutrino Observatory consists of 1,000 tons of heavy water more than 2,000 m below the surface. It detects neutrinos from the Sun, where only electron-neutrinos are produced. The reactions between neutrinos and the heavy water in the tank yielded the possibility to separately measure both electron-neutrinos alone and all three types of neutrinos combined. It was discovered that the electron-neutrinos were fewer than expected, while the total number of all three types of neutrinos combined matches the expectations. The conclusion is that some of the electron-neutrinos have changed into another identity.
Sudbury Neutrino Observatory is a cooperation of scientists from Canada, Germany, Portugal, UK and USA. Germany is represented with the group of Prof. Kai Zuber from the HAP partner Technische Universität Dresden.
|
Super-Kamiokande
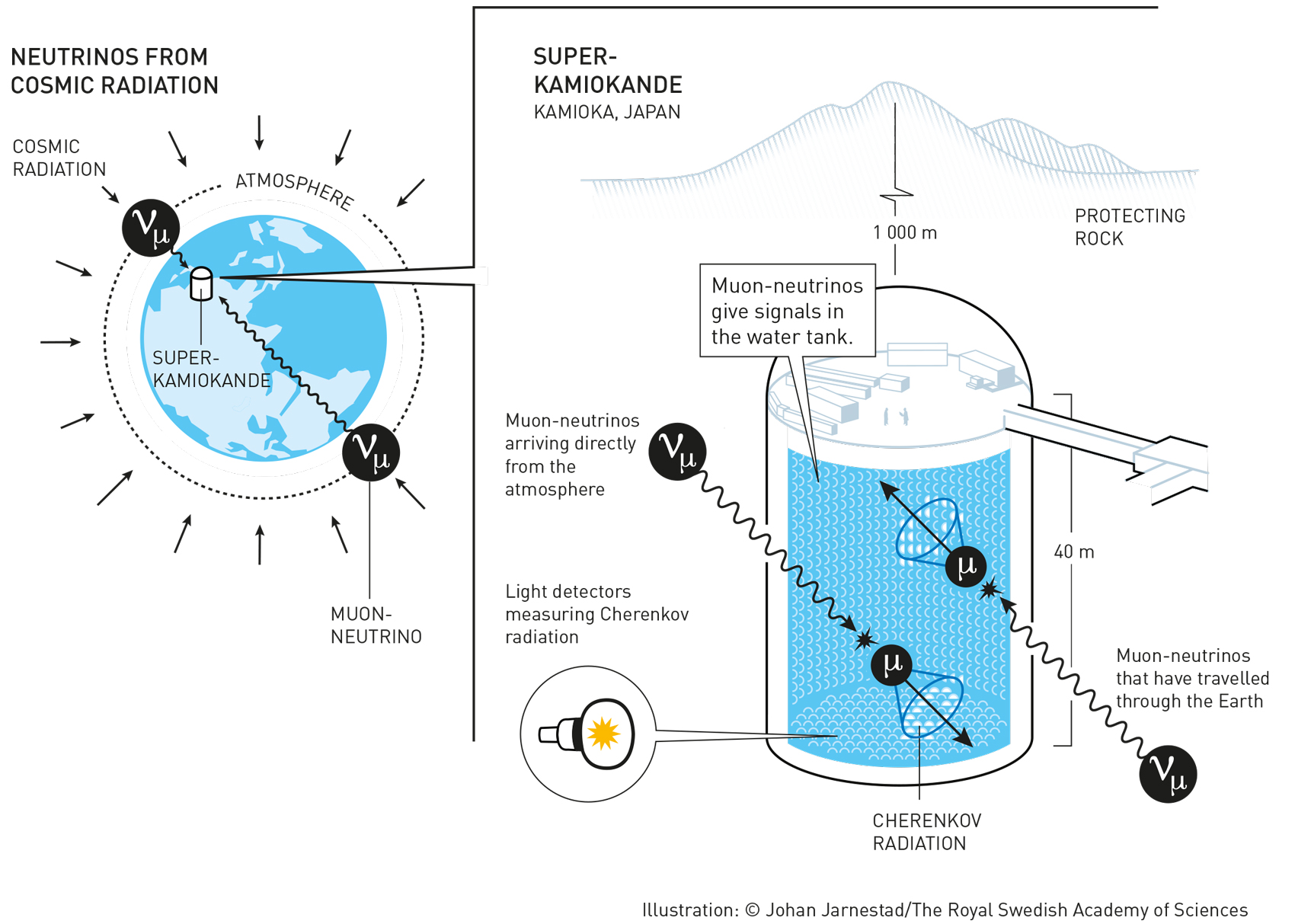
Super-Kamiokande, a detector built 1,000 metres below the Earth’s surface detecting atmospheric neutrinos.
Super-Kamiokande is a gigantic detector built 1,000 metres below the Earth’s surface. It consists of a tank, 40 metres high and as wide, filled with 50,000 tonnes of water. It detects atmospheric neutrinos. When a neutrino collides with a water molecule in the tank, a rapid, electrically charged particle is created. This generates Cherenkov radiation that is measured by the light sensors. The shape and intensity of the Cherenkov radiation reveals the type of neutrino that caused it and the direction from where it came. The muon-neutrinos that arrived at Super-Kamiokande from above were more numerous than those that travelled through the entire Earth. This indicated that the muon-neutrinos that travelled longer had time to change into another identity on their way.
Super-Kamiokande is a cooperation of scientists from Japan, Canada, Korea, Spain and USA.
|
30.09.2015 - Senior Fellows of the Helmholtz Alliance for Astroparticle Physics 2015
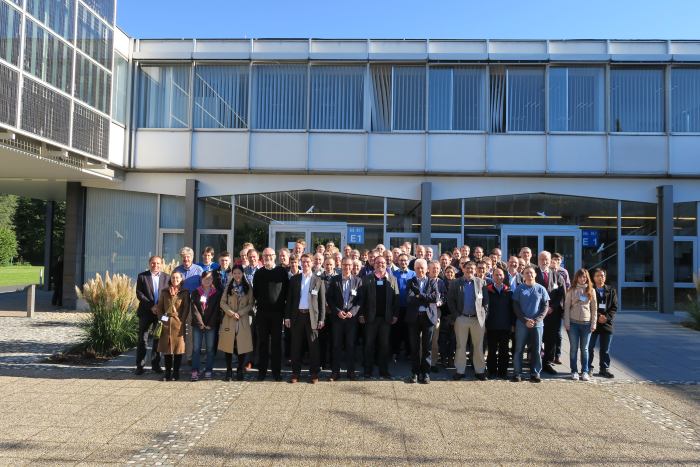
Group picture of the first annual meeting Matter and the Universe
(credit: HAP/A.Chantelauze)
On September 29-30 took place the annual meeting of the Helmholtz Programme Matter and the Universe at Forschungszentrum Jülich. This first annual meeting of the programme at the Research Center Jülich brought together the different programme communities with activities in elementary particle physics, hadron physics, nuclear physics and astroparticle physics in order to strengthen the co-operation across the different programme topics.
|
|
|
|
Left: Dr. Keilhauer (HAP spokesperson) and Prof. Dr. Grupen, Right: Dr. Keilhauer and Prof. Dr. Meyer
(credit: HAP/A.Chantelauze)
|
During the meeting also took place the Senior Fellow award ceremony of the Helmholtz Alliance for Astroparticle Physics. The title of Senior Fellow of the Helmholtz Alliance for Astroparticle Physics in 2015 has been awarded to:
- Prof. Dr. Claus Grupen (University of Siegen),
- Prof. Dr. Hinrich Meyer (University of Wuppertal).
The two retired scientists are awarded a Senior Fellowship, travel funds for conferences and schools associated with HAP as well as funding opportunities for scientific projects. Thus, the recognized scientists can continue to be involved in current research and to share their experience and knowledge within the Alliance.
Press release:
Uni. Siegen,
Uni. Wuppertal
30.09.2015 - Erste Gammastrahlenbilder von neuer H.E.S.S.-Kamera
Die neue Kamera, montiert am Teleskop.
(Bild: Stefan Klepser/DESY)
Am Gammastrahlen-Observatorium H.E.S.S. in Namibia ist die erste von vier neuen Gammastrahlenkameras in Betrieb gegangen. Ein zehnköpfiges Team vom DESY-Standort Zeuthen hatte die Kamera im Juli montiert und dabei 200 Elektronikboards sowie ein neues Lüftungssystem installiert. Nach Anbindung an das Netzwerk und das Datennahmesystem von H.E.S.S. (High Energy Stereoscopic System) zeichnete die neue, schnellere Kamera jetzt die ersten kosmischen Gammastrahlen auf.
Mit H.E.S.S. beobachten Physiker kosmische Gammastrahlung, die uns von den energiereichsten Objekten im Universum erreicht: Supernova-Explosionen, aktive Galaxien mit gigantischen Schwarzen Löchern im Zentrum, ausgebrannte Riesensonnen und weitere exotische Himmelsobjekte. Die Kameras der H.E.S.S.-I-Teleskope sind seit 2003 in Betrieb und leiden daher zunehmend an Alterung und den Folgen der harschen Umgebungsbedingungen des Khomas-Hochlandes. In den vergangenen zwei Jahren wurde daher eine Rundumerneuerung der Elektronik und Belüftung dieser technologisch anspruchsvollen Geräte entwickelt. An einem Teststand bei DESY in Zeuthen wurden alle neue Komponenten vorab getestet.
„Die Tests im Labor waren sehr wichtig, sie stellen letztlich aber nie vollständig die realen Bedingungen in der Wüste nach", resümiert Projektleiter Stefan Klepser (DESY). „Ich bin daher sehr froh, dass wir tatsächlich alle Installationen innerhalb der veranschlagten drei Wochen geschafft haben." An der Installation in Namibia haben 20 Ingenieure und Wissenschaftler teilgenommen, die Hälfte davon von DESY, dazu H.E.S.S.-Kollegen aus Windhoek, Heidelberg, Paris und Berlin.
Die neue Kameraelektronik kann Lichtblitze, die teils nur 20 bis 30 Lichtquanten enthalten und wenige Nanosekunden andauern, in Echtzeit registrieren und digitalisieren. „Das Design der Elektronikboards war eine große Herausforderung, viele der Leiterplatten sind mit über 1 000 Einzelbauteilen bestückt", erläutert Elektronik-Entwickler Holger Leich. „Dank der Fertigung bei DESY in Hamburg konnten wir unsere Qualitätsansprüche jedoch 1:1 umsetzen."
Die bloße Hardware-Installation ist auf dem Weg zur vollständigen Integration im Teleskopsystem jedoch nur der halbe Weg. „Die Software der neuen Kamera wurde komplett neu entwickelt", berichtet Gianluca Giavitto aus Zeuthen, der die Zusammenführung der Systeme vor Ort über mehrere Wochen geleitet hat. „Da die ganze Kamera über Ethernet funktioniert, haben wir aktuelle Technologien verwendet, die teils auch bei Facebook und Google im Einsatz sind. Das hat die Entwicklung beschleunigt und vereinfacht, und die Stabilität des Systems verbessert."
Bis zur vollständigen Eichung und Analyse der neuen Kamera werden noch einige Monate vergehen. „Die grundlegende Funktionalität ist mit diesen Bildern jedoch bewiesen, und die Produktion der anderen drei Kameras, die 2016 installiert werden sollen, kann beginnen", sagt der Leiter des DESY-Standorts Zeuthen, Christian Stegmann. Zuversichtlich sind auch die Entwickler des analogen Auslesechips NECTAr, der in der Kamera erstmalig Verwendung findet. Die neue H.E.S.S.-Kamera gilt als proof-of-principle für die neue Chiptechnologie, die am IRFU in Saclay (Frankreich) für das geplante Cherenkov Telescope Array (CTA) entwickelt wurde.
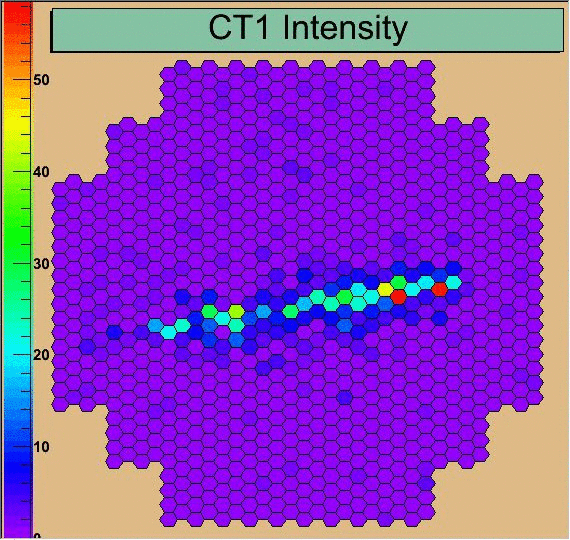
Einige der ersten Gamma-Bilder, die von der Kamera aufgezeichnet wurden.
(Bild: The H.E.S.S. Collaboration)
Pressemitteilung: DESY
01.07.2015 - Double Doctoral Degree of KIT and UNSAM
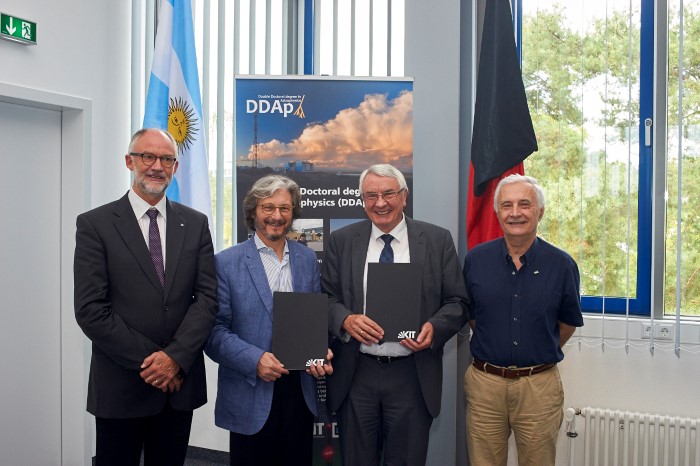
From left to right: Johannes Blümer (spokesperson of KCETA), Carlos Ruta (rector of UNSAM),
Detlef Löhe (vice-President of KIT), Alberto Etchegoyen (director of ITeDA)
(credit: KIT)
On Sept 1st, the Karlsruhe Institute of Technology (KIT) and the Universidad Nacional de San Martin (UNSAM) celebrated their Double Doctoral degree in Astrophysics (DDAp), which started this year. German and Argentinean scientists, academic authorities, professors and students were gathered to officially kick-off the double degree at KIT.
The programm of the day was to present the DDAp to the future graduate students and to highlight the long lasting friendship between UNSAM and KIT. Detlef Löhe, Vice-President of KIT and Carlos Ruta, Rector of UNSAM welcomed the participants and presented their respective university, while Johannes Blümer, spokesperson of the KIT Center Elementary Particle and Astroparticle Physics (KCETA) and Alberto Etchegoyen, director of the Institute of Technology and Astroparticle Detection (ITeDA), Buenos Aires, highlighted the close connection between KIT and UNSAM over the last decades, especially within the Pierre Auger Observatory, and the huge potential of cooperation for the future.
The celebrations continued with an overview about the development of nuclear physics at Forschungszentrum Karlsruhe by Manfred Popp. Then Frank Schröder described the concept and the organisation of the DDAp and Ewa Holt, one of the first DDAp participants, gave her impressions on her first half year in Buenos Aires within the frame of the programme. It was followed by a talk of Bernd Müller-Jacquier (Universität Bayreuth), who described the linguistic problems that could occur when working in intercultural environments. Finally Alan Watson (University of Leeds), Spokesperson Emeritus of the Pierre Auger Observatory, presented a history of research on high-energy particle physics.
The Double Doctoral degree in Astrophysics (DDAp) is jointly offered by the two HAP-partners the Physics Department at the Karlsruhe Institute of Technology (KIT) in Germany and the Institute of Technology in Detection and Astrophysics (ITeDA)
at the Universidad Nacional de San Martín (UNSAM) in Buenos Aires, Argentina. Doctoral researchers participating in the DDAp work on joint research projects in astroparticle physics, e.g., related to the Pierre Auger Observatory ,
and spend at least one year in the other country. The doctoral researchers have to follow the rules of both universities and a specific cooperation agreement. The research project is complemented by a lecture program covering astrophysics, astroparticle and particle physics as well as language courses. At the conclusion of a project after typically three years, KIT and UNSAM, will conduct a joint examination and both will issue their doctoral degree.
Over the last years, doctoral researchers already beneficiated of the opportunity to spend part of their doctoral work abroad either at KIT or at UNSAM. These research periods will now cumulate for a double degree. Last May, similar kick-off celebrations already took place at UNSAM. Eight doctoral researches are already participating in the double degree programme.
The DDAp is supported by the German Argentinean University Center (CUAA-DAHZ), BMBF and DAAD in Germany, and Ministerio de Educación and Ministerio de Cienca, Tecnologia e Innovación Productiva in Argentina.
Double Doctoral degree in Astrophysics
Coordinator of the DDAp at KIT is Prof. Blümer.
Questions regarding the DDAp can also be addressed to Dr. Frank Schröder.
Coordinator of the DDAp at ITeDA/UNSAM is Prof. Etchegoyen.
More pictures: indico website of the Kick-Off event
31.07.2015 - CTA Consortium Spokesperson Prof. Werner Hofmann Wins Yodh Prize
|
|
Werner Hofmann, Consortium Spokesperson
of the Cherenkov Telescope Array
(credit: WeAreTheNight.com / Igor Roelofsen)
|
Picture artist of the future CTA array
(credit: IEEEC)
|
Prof. Werner Hofmann, CTA Consortium Spokesperson, was awarded the Yodh Prize "for his outstanding leadership in the field of high-energy gamma-ray astronomy" at the 34th International Cosmic Ray Conference in The Hague, The Netherlands, where CTA Consortium members willcontribute eight talks and 59 posters. The Yodh Prize recognizes a scientist whose research career has had a major impact on the understanding of cosmic rays. It was endowed by Gaurang and Kanwal Yodh to the University of California Irvine Foundation in 1998.
Prof. Hofmann was selected by an international committee of distibguished scientists in the field of cosmic rays and astroparticle physics. The award is administered through the Commission on Astroparticle Physics of the Unternational Uniuon of Pure and Applied Physics (IUPAP) and is presented every two years at the ICRC. In addition to a cash prize, the winner is invited to present a Department of Physics and Astronomy Colloquium at the University of California, Irvine.
Press release: CTA
17.07.2015 - Neutrino-Dissertationspreis: Zwei Gewinner bei DESY
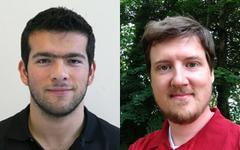
GNN-Preisträger Juan Pablo Yáñez (l.) und Jakob van Santen
|
Zweimal Grund zur Freude in der IceCube-Gruppe bei DESY: Juan Pablo Yáñez und Jakob van Santen, beide am DESY-Standort in Zeuthen, haben zwei der drei Dissertationspreise des Global Neutrino Network GNN erhalten. Beide haben mit Daten des weltweit größten Neutrinodetektors IceCube gearbeitet, der in das ewige Eis der Antarktis eingeschmolzen ist.
Juan Pablo Yáñez hat seine Arbeit „Measurement of neutrino oscillations in atmospheric neutrinos with the IceCube DeepCore detector“ als Student der Humboldt Universität bei DESY geschrieben. Er hat Neutrinos untersucht, die mit IceCube aufgezeichnet wurden. Dabei gelang es ihm, den erlaubten Bereich für zwei Parameter einzugrenzen, von denen die gegenseitige Umwandlung verschiedener Neutrinosorten entscheidend abhängt – auch Neutrinooszillation genannt. Damit hat er bewiesen, dass ein Detektor in natürlichem Eis in der Lage ist, Präzisionsresultate auf dem Gebiet der Neutrinophysik zu liefern. Die Resultate wurden in „Physical Review D“ 91, 072004 (2015) veröffentlicht (siehe auch arXiv:1410.7227).
Jakob van Santen hat seine Dissertation an der University of Wisconsin verfasst und konnte danach als Postdoc für die Zeuthener Gruppe gewonnen werden. In seiner Arbeit „Neutrino Interactions in IceCube above 1 TeV: Constraints on Atmospheric Charmed-Meson Production and Investigation of the Astrophysical Neutrino Flux with 2 Years of IceCube Data taken 2010-2012“ hat er das Energiespektrum und die Winkelverteilung von hochenergetischen Neutrinos studiert, die mit IceCube registriert wurden. Seine Arbeit hat neue, wichtige Informationen zu den kürzlich entdeckten Neutrinos astrophysikalischen Ursprungs geliefert. Die Untersuchung wurde in „Physical Review D“ 91, 022001 (2015) publiziert (siehe auch arXiv:1410.1749).
Das Global Neutrino Network wurde am 15. Oktober 2013 gegründet und hat sich zum Ziel gesetzt, die weltweiten Aktivitäten zu Bau, Betrieb und Auswertung großer Neutrinoteleskope in tiefem Wasser und Eis zu koordinieren. Der GNN-Dissertationspreis hat in diesem Jahr Premiere. Er wird an Kandidaten verliehen, die sichtbar zum Erfolg ihres Projekts beigetragen und eine hervorragende Dissertation geschrieben haben.
Pressemitteilung: DESY
|
16.07.2015 - Paranal und La Palma erste Wahl als Standorte für CTA
|
|
|
|
Die Mitglieder des CTA Resource Boards besuchten
während ihres Treffens in Zeuthen auch
den Teleskopprototypen in Berlin.
(Quelle: DESY)
|
Paranal und La Palma erste Wahl als Standorte für CTA
(Quelle: CTA-Kollaboration)
|
Auf ihrem Treffen am 15. und 16. Juli in Zeuthen beschloss das CTA Resource Board, in detaillierte Vertragsverhandlungen für den nördlichen und den südlichen Standort des Gamma-Observatoriums CTA einzutreten. Als südlicher Standort Paranal in Chile ausgewählt, als Standort der nördlichen Hemisphäre die spanische Atlantikinsel La Palma.
Das CTA Resource Board, das aus Vertretern von Ministerien und Förderorganisationen aus Brasilien, Tschechien, Frankreich, Deutschland, Italien, Japan, Namibia, den Niederlanden Österreich, Polen, Südafrika, Spanien, Schweiz und Großbritannien besteht, hatte sich vorher intensiv beraten und die Gegebenheiten an den in Frage kommenden Orten abgewogen. Dabei ging es insbesondere darum, für den Bau und den späteren wissenschaftlichen Betrieb der Anlage das Optimum zu erzielen.
„Alle Standorte, über die wir in der finalen Runde beraten haben, haben sehr hochwertige und engagierte Bewerbungen abgegeben“, sagte CTA-Sprecher Werner Hofmann (MPI Heidelberg). „Es ist dem Resource Board sehr schwergefallen, sich für diese zwei Standorte zu entscheiden.“ „Diese Entscheidung ist ein wichtiger Schritt in Richtung der Realisierung des CTA-Observatoriums“, sagte Beatrix Vierkorn-Rudolph (Bundesministerium für Bildung und Forschung), die den Vorsitz des CTA Resource Boards innehat.
„Mit diesen wegweisenden Entscheidungen zu den endgültigen Standorten für CTA im Rücken können wir uns jetzt darauf konzentrieren, zusammen mit allen CTA-Partnern das technisch und wissenschaftlich beste Gamma-Observatorium zu bauen, das es je gegeben hat“; ergänzt Christian Stegmann (DESY).
Paranal, Chile (Quelle: ESO)
Der jetzt gewählte Standort in der südlichen Hemisphäre liegt in der Atacama-Wüste und ist nur etwa 10 Kilometer vom bereits existierenden ESO-Standort Paranal entfernt. Damit liegt er nicht nur an einem der trockensten Orte auf der Erde – ein Paradies für astronomische Beobachtungen –, sondern die Wissenschaftler können voraussichtlich sehr von der bereits vorhandenen ESO-Infrastruktur profitieren.
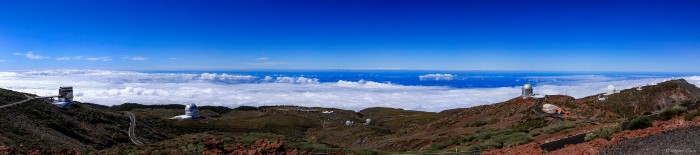
La Palma, Spanien (Quelle: Miguel Claro)
Der nördliche Standort auf der Kanareninsel La Palma liegt in unmittelbarer Nähe der MAGIC-Teleskope und bietet ebenfalls klare Höhenluft über lange Perioden des Jahres.
Das CTA-Observatorium soll in klaren Nächten das Cherenkov-Licht auffangen, das entsteht, wenn hochenergetische Gammastrahlung auf die irdische Atmosphäre trifft. Es soll aus etwa 100 verschieden großen Cherenkov-Teleskopen auf der Südhalbkugel und etwa 20 auf der Nordhalbkugel der Erde bestehen. Damit wird eine optimale Abdeckung des Nachthimmels erreicht. Orte in Namibia und Mexiko, die sich ebenfalls als Standorte beworben hatten, werden weiterhin als mögliche Alternativen vorgesehen. Nach endgültigem Abschluss der Standortverhandlungen ist der Baubeginn der ersten CTA-Teleskope für 2016 vorgesehen.
Pressemitteilung: DESY
30.04.2015 - Prestigious award to IceCube pioneer
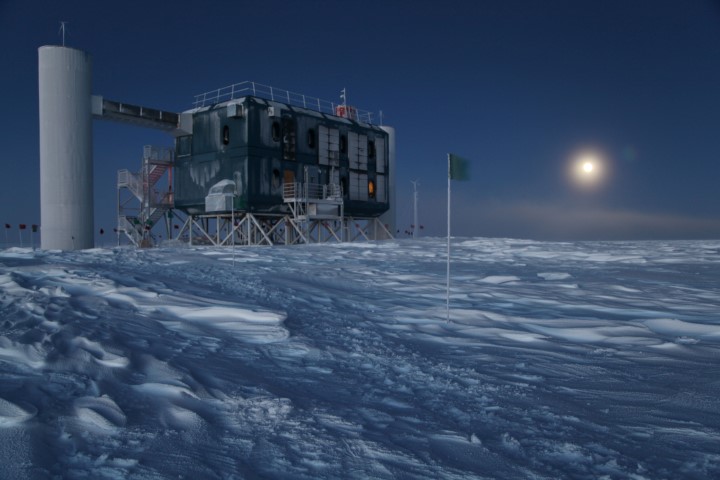
Francis Halzen, principal investigator of the IceCube - South Pole Neutrino Observatory
The European Physical Society (EPS) awards its 2015 Giuseppe and Vanna Cocconi Prize for an outstanding contribution to Particle Astrophysics and Cosmology to IceCube
principal investigator Francis Halzen (University of Wisconsin, Madison). The prestigious price acknowledges "his visionary and leading role in the detection of very high-energy extraterrestrial neutrinos, opening a new observational window on the Universe".
"I feel very honored to receive this prize," says Halzen. "But I understand it firstly as recognition of a great collective effort of the whole IceCube collaboration." This is the second important international appreciation following IceCube's 2013 discovery of cosmic neutrinos, the first being the award "Breakthrough of the Year 2013" by the journal Physics World.
The IceCube physicists from Germany share Halzen's way since more than 20 years and hosted him for frequent working stays in Zeuthen and elsewhere. HAP members played a significant role in breaking the wall to the high-energy neutrino Universe.
Christian Spiering, DESY
30.04.2015 - Ergebnisse des Neutrino-Observatoriums IceCube eröffnen neue Perspektiven für die Teilchenphysik

In der IceCube-Zentrale an der Scott-Amundsen-Station
am Südpol laufen die Detektordaten zusammen.
(Bild: Felipe Pedreros, IceCube/NSF)
Das IceCube-Observatorium am Südpol hat vermessen, auf welche Art und Weise Neutrinos, eine Sorte geisterhafter Elementarteilchen, ihre Identität verändern, wenn sie durch den Erdball und die irdische Atmosphäre fliegen. Die Messung dieser Neutrino-Oszillationen, die erstmals 1998 vom Kamiokande-Experiment in Japan beobachtet wurden, eröffnet neue Perspektiven für die Teilchenphysik, und das mit einem Detektor, der ursprünglich für ganz andere Zwecke gebaut wurde, nämlich zur Entdeckung von Neutrinos aus weit entfernten kosmischen Quellen. „Wir freuen uns sehr, dass wir mit IceCube und dem integrierten DeepCore-Detektor Neutrino-Oszillationen mit hoher Präzision bestimmen können", sagt Olga Botner, Sprecherin des IceCube-Experiments. „DeepCore wurde auf Initiative des erst kürzlich verstorbenen Per Olof Hulth entwickelt, um die Energieschwelle von IceCube deutlich herabzusetzen. Die Ergebnisse zeigen, dass IceCube dazu beitragen kann, die Oszillationsparameter sehr genau zu bestimmen; ein guter Grund, die Pläne für PINGU voranzutreiben, die IceCube-Erweiterung zur Messung von Neutrinoeigenschaften."
„IceCube registriert jedes Jahr mehr als hunderttausend atmosphärische Neutrinos. Die meisten davon sind Myon-Neutrinos, die durch die Wechselwirkung von schnellen kosmischen Teilchen mit der Atmosphäre entstehen", sagt DESY -Physiker Rolf Nahnhauer. Mit dem DeepCore Detektor im Zentrum von IceCube können Neutrinos mit Energien bis hinunter zu 10 Gigaelektronenvolt (GeV) nachgewiesen werden. „Aufgrund unseres Wissens über Neutrino-Oszillationen müsste IceCube weniger Myon-Neutrinos mit Energien um 25 GeV registrieren, wenn sie nach Durchqueren des Erdballs IceCube erreichen", erklärt Nahnhauer weiter. „Der Grund für das Fehlen der Myon-Neutrinos liegt in deren Umwandlung in andere Neutrino-Sorten." IceCube-Forscher nutzten Daten von Myon-Neutrinos von der Nordhalbkugel, in einem Energiebereich von wenigen GeV bis rund 50 GeV, die im Zeitraum Mai 2011 bis April 2014 genommen wurden. Es wurden etwa 5 200 Ereignisse gefunden, wesentlich weniger als die 7 000, die man unter Annahme von nicht stattfindenden Oszillationen erwartet hätte.
Noch heute gehören Neutrinos zu den rätselhaftesten Elementarteilchen. 1930 vom österreichischen Physiker Wolfgang Pauli vorhergesagt, gelang deren experimenteller Nachweis erst 25 Jahre später. „Neutrinos sind geisterhafte Teilchen", so Olga Botner, „sie fliegen durch Materie aller Art und durch den gesamten Erdball, ohne mit irgendetwas zu reagieren." Die Physiker haben jedoch immer feinere Messinstrumente gebaut, um die Rätsel der extrem leichten Teilchen zu entschlüsseln. Eines der überraschenden Ergebnisse war, dass die drei Neutrinosorten Elektron-, Myon- und Tau-Neutrino ihre Identität ändern können, indem sie von einer zur anderen Neutrinosorte wechseln. Dieses Phänomen bezeichnet man als Neutrino-Oszillation. „Neutrino-Oszillationen sind aber nur möglich, wenn Neutrinos eine Masse haben", erklärt Nahnhauer. „Im Standardmodell der Teilchenphysik werden Neutrinos jedoch als masselos angenommen." Die Oszillationsstärke und die Strecke, auf der diese Oszillation stattfindet, wird von zwei Parametern bestimmt: dem sogenannten Mischungswinkel und der Massendifferenz der einzelnen Sorten. Die Werte dieser Parameter wurden eingegrenzt durch präzise Messungen von Neutrinos aus der Sonne, der Atmosphäre, Kernkraftwerken und Teilchenbeschleunigern.
Das Neutrino-Observatorium IceCube am Südpol, das das antarktische Eisschild als Detektormaterial nutzt, hat bereits seine Leistungsfähigkeit als Instrument zur Erforschung des Universums mit Hilfe von Neutrinos bewiesen. Mehr als 5 000 optische Sensoren, auf einen Kubikkilometer Eis verteilt, registrieren die äußerst seltenen Kollisionen von Neutrinos mit dem Eis. Vor knapp zwei Jahren verkündeten die IceCube-Physiker die Entdeckung der ersten hochenergetischen Neutrinos aus dem Kosmos. Diese bahnbrechende Entdeckung wurde vom Wissenschaftsmagazin „Physics World" zum „Durchbruch des Jahres" gekürt.
Visualisierung der Digitalen Optischen Module (DOM)
von IceCube im antarktischen Eis.
(Bild: Jamie Yang, IceCube Collaboration)
Jetzt hat IceCube bewiesen, dass nicht nur Spitzenergebnisse für die Astrophysik, sondern auch äußerst wichtige Ergebnisse für die Teilchenphysik möglich sind. Die neuen Messungen der IceCube-Kollaboration erlauben eine wesentlich verbesserte Bestimmung der Neutrino-Oszillationsparameter. Die Resultate wurden jetzt vom Wissenschaftsmagazin „Physical Review D" zur Veröffentlichung angenommen.
Die im Zeitraum von drei Jahren an IceCube gelieferten Daten waren ähnlich präzise wie diejenigen, die in 15 Jahren am Super-Kamiokande gemessen wurden. Anders als Super-Kamiokande, mit seinem 50000-Tonnen-Tank hochreinen Wassers, nutzt IceCube das ewige Eis des Südpols als Detektormaterial. Das 500 Mal größere Beobachtungsvolumen liefert höhere Ereignisstatistiken in kürzerer Zeit. „Sowohl Super-Kamiokande als auch IceCube nutzen den gleichen ‚Teilchenstrahl‘ aus atmosphärischen Neutrinos, aber mit unterschiedlichen Energien. Wir erreichen dabei eine vergleichbare Präzision der messbaren Oszillationsparameter", sagt DESY-Postdoc Pablo Yanez, verantwortlicher Autor des wissenschaftlichen Beitrags. „Die Ergebnisse aus IceCube-Daten haben zurzeit zwar noch größere Messfehler als die extrem präzisen Neutrinostrahlexperimente MINOS und T2K; IceCube wird jedoch weiterhin Daten nehmen, und mit Verbesserung der Analysen hoffen wir, bald aufholen zu können", ergänzt Yanez.
Zurzeit ist eine Erweiterung des IceCube Detektors namens PINGU (Precision IceCube Next Generation Upgrade) in Planung. Eine wesentlich höhere Dichte der optischen Module im gesamten zentralen Bereich soll die Empfindlichkeit für die Erforschung verschiedener fundamentaler Fragen im Zusammenhang mit Neutrinos erhöhen.
"In erster Linie wollen wir die sogenannte Neutrino-Massenhirarchie messen, das heißt, ob es zwei schwerere und ein leichtes Neutrino gibt, oder ob es genau umgekehrt ist", erklärt Nahnhauer. „Das ist wichtig, um zu verstehen, wie Neutrinos Masse erhalten, es hat aber auch eine erhebliche Bedeutung bei der Entwicklung des Kosmos. Die aktuellen Ergebnisse liefern eine wichtige experimentelle Bestätigung dafür, dass unsere Ideen funktionieren."
Pressemitteilung: DESY
Publikation: Measurement of the Atmospheric νe Spectrum with IceCube, IceCube Collaboration, submitted to Physical Review D (arXiv:1504.03753)
Mehr lesen: CERN Courier, IceCube website
29.04.2015 - Kosmische Gammablitze als Teilchenbeschleuniger
|
|
|
|
Künstlerische Darstellung eines Gammastrahlenblitzes.
(Bild: NASA)
|
Das Plasma wird in Form unterschiedlich schneller Schalen ausgeworfen. Kollidieren diese Schalen, werden dabei Teilchen beschleunigt.
(Illustration: Mauricio Bustamante/DESY)
|
Eine neue Studie bietet detaillierte Einblicke in die gewaltigsten Explosionen des Universums, sogenannte Gammastrahlenblitze. Die Simulation beschreibt die Beschleunigung kosmischer Teilchen in diesen seltenen Explosionen genauer als bisher und kann scheinbare Widersprüche aufklären, die sich aus astrophysikalischen Beobachtungen ergeben haben. Forscher von DESY und aus den USA stellen ihre Arbeit im Fachblatt „Nature Communications“ vor.
Zu Gammastrahlenblitzen kommt es, wenn extrem große Sterne als Supernova explodieren. Diese Explosionen sind nahezu durch das gesamte Universum zu sehen, bis in Milliarden Lichtjahre Entfernung. Die starken Magnetfelder der explodierenden Riesensonnen führen den größten Teil der Explosionsenergie in zwei Strahlen aus elektrisch geladenem Gas, die sich von den Polen des Sterns ins All hinaus erstrecken. Diese sogenannten Plasma-Jets sind starke natürliche Teilchenbeschleuniger.
Forscher nehmen an, dass aus den Plasma-Jets von Gammastrahlenblitzen zumindest ein Teil der energiereichen elektrisch geladenen Teilchen stammt, die unablässig in die Erdatmosphäre prasseln. Dieser Teilchenhagel aus dem All wird als kosmische Strahlung bezeichnet und besteht hauptsächlich aus Wasserstoffatomkernen (Protonen). Manche Teilchen der kosmischen Strahlung haben zehn Millionen Mal mehr Energie als die Protonen im derzeit stärksten irdischen Beschleuniger, dem Large Hadron Collider (LHC) am Forschungszentrum CERN bei Genf.
Sind die Gammastrahlenblitze allerdings tatsächlich eine nennenswerte Quelle kosmischer Strahlung, so erwarten die Forscher von ihnen aus physikalischen Gründen auch eine große Anzahl leichter Elementarteilchen namens Neutrinos. Das Südpol-Neutrinoteleskop IceCube, bei dem DESY der größte europäische Partner ist, sucht nach genau solchen energiereichen kosmischen Neutrinos, konnte von Gammastrahlenblitzen bislang jedoch keine nachweisen. Den bisherigen Messungen zufolge erreichen uns von den gewaltigen Explosionen mindestens zehnmal weniger Neutrinos als erwartet. „Das wirft neue Fragen an die Theorie auf“, sagt DESY-Forscher Walter Winter, Mitautor der Studie. „Möglicherweise war unser Bild von Gammastrahlenblitzen bisher zu einfach.“
Neutrinos entstehen vor allem in vergleichsweise geringer Entfernung von der Quelle,
die Teilchen der geladenen kosmischen Strahlung in mittlerem Abstand
und Gammastrahlung in größerer Distanz.
(Illustration Mauricio Bustamante/DESY)
Bisherige Modelle waren davon ausgegangen, dass Protonen, Neutrinos und die Gammastrahlung am selben Ort in den Plasma-Jets entstehen. Das Team von Astroteilchen-Theoretikern, zu dem neben Winter auch Mauricio Bustamante von der Ohio State University sowie Philipp Baerwald und Kohta Murase von der Pennsylvania State University zählen, hat jetzt ein dynamischeres Bild der Gammablitze entwickelt: Das Plasma wird demnach schalenförmig mit unterschiedlichen Geschwindigkeiten in den Weltraum geschleudert. Diese Schalen kollidieren in größerem Abstand von ihrer Quelle miteinander, wobei dann die Teilchen beschleunigt werden.
Dieser Ansatz kann die beobachtete starke Schwankung der Helligkeit von Gammastrahlenblitzen gut erklären. Eine Konsequenz dieses Modells ist zudem, dass verschiedene Emissionen wie Neutrinos, Protonen und Gammastrahlung in ganz unterschiedlichen Regionen des Plasma-Jets erzeugt werden. Damit lässt sich unter anderem erklären, warum nicht die erwartete Menge Neutrinos beobachtet werden konnte. „Wir erwarten, dass die nächste Generation von Neutrinoteleskopen wie IceCube-Gen-2 empfindlich genug sein wird für diesen minimalen Neutrinofluss, den wir vorhersagen“, betont Bustamante. Im Vergleich zu früheren Ansätzen hängt diese Abschätzung nur wenig von den Parametern des zugehörigen Gammastrahlenblitzes ab.
Pressemitteilung: DESY
Publikation: Neutrino and cosmic-ray emission from multiple internal shocks in gamma-ray bursts, Mauricio Bustamante, Philipp Baerwald, Kohta Murase & Walter Winter, Nature Communications 6, Article number: 6783, 2015 (arXiv:1409.2874)
22.04.2015 - EU-Millionenförderung für Forschung zu Neutrinos

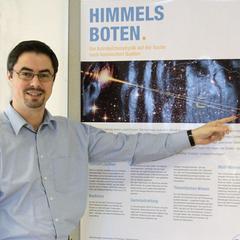
Walter Winter bekommt den ERC Grant
für sein Projekt NeuCos.
|
Der Europäische Forschungsrat (European Research Council, ERC) fördert ein Forschungsprojekt von DESY-Wissenschaftler W. Winter mit sogenanntem ERC Consolidator Grant. Walter Winter aus Zeuthen wird für fünf Jahre mit rund 1,75 Millionen Euro für sein Projekt „NeuCos – Neutrinos and the origin of the cosmic rays" unterstützt. Der hochdotierte ERC Consolidator Grant wird im Rahmen des EU-Förderprogramms „Horizon 2020" an exzellente, hoch talentierte Wissenschaftler zur Förderung ihrer unabhängigen und selbstständigen Forschungstätigkeit vergeben.
Walter Winter, der auch an der Humboldt-Universität zu Berlin (HU Berlin) lehrt, will in seinem Projekt die kosmische Strahlung genauer erforschen. Die Quellen dieser vor über 100 Jahren entdeckten Strahlung geben nach wie vor Rätsel auf. Es müssen gigantische Teilchenbeschleuniger sein, der genaue Ursprung ist jedoch besonders bei den höchsten Energien unbekannt. Mögliche Kandidaten für die Beschleuniger dieser kosmischen Teilchen sind Gammastrahlungsblitze oder aktive Galaxienkerne.
|
Ein weiteres Rätsel der kosmischen Strahlung ist deren chemische Zusammensetzung: Daten des Pierre Auger Observatorium deuten auf schwerere Atomkerne bei den höchsten Energien hin – ein Ergebnis, das weitere Fragen aufwirft. Gleichzeitig haben die Beobachtungen höchstenergetischer Neutrinos mit dem Neutrinoteleskop IceCube die Hochenergie-Astrophysik im Jahr 2013 revolutioniert. Zum ersten Mal wurde neben Photonen und kosmischer Strahlung ein weiteres Puzzleteil für die Natur der kosmischen Beschleuniger gefunden.
Ein Ziel des Forschungsprojektes „Neutrinos and the origin of the cosmic rays" ist es, eine gemeinsame Grundlage für diese verschiedenen Beobachtungen zu suchen. Dafür will Winter eine multidisziplinäre Theorie-Arbeitsgruppe mit Expertise aus der Astrophysik und Teilchenphysik gründen. Sie soll theoretische Modelle der Quellen schwererer Kerne entwickeln, um aus den Beobachtungsdaten der verschiedenen kosmischen Botschafter neue Hinweise für den Ursprung der kosmischen Strahlung zu erhalten. Ein weiteres Ziel ist es, die teilchenphysikalischen Eigenschaften der Neutrinos mit Hilfe zukünftiger Neutrinoteleskope zu untersuchen.
Pressemitteilung: DESY
23.03.2015 - Two new associated partners for HAP

The Helmholtz Alliance for Astroparticle Physics welcomes with great pleasure two new associated partners from Amsterdam, Netherlands:
- the GRavitation and AstroParticle Physics in Amsterdam (GRAPPA) and
- the National Institute for Subatomic Physics (Nikhef).
GRAPPA is a new center of excellence of the University of Amsterdam. It consists of faculty member and outstanding scientists from the Institute for High Energy Physics (IHEF), the 'Anton Pannekoek' Institute (API) and the Institute for Theoretical Physics Amsterdam (ITFA).
The aim of the new center is to bring together theoretical physicists, astronomers and particle physicists, in order to answer some of the most profound questions in Particle Astrophysics and Cosmology.
Nikhef is a partnership between the Foundation for Fundamental Research on Matter (FOM) and four universities: Radboud University Nijmegen, University of Amsterdam, Utrecht University and VU University Amsterdam. The mission of Nikhef is to study the interactions and structure of all elementary particles and fields at the smallest distance scale and the highest attainable energy.
Two complementary approaches are followed: Accelerator-based particle physics and Astroparticle physics.
Nikhef coordinates and leads the Dutch experimental activities in these fields. The research at Nikhef relies on the development of innovative technologies.
23.03.2015 - HAP Annual Member Board Meeting
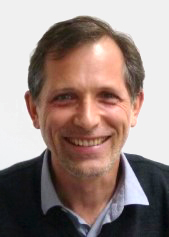
Bianca Keilhauer (KIT) and Josef Jochum (Uni Tübingen) were respectively
elected and reelected Scientific Coordinator and Spokespersons of HAP.
On March 23rd, the Helmholtz Alliance for Astroparticle Physics held its annual HAP Member Board meeting. This meeting was devoted to many elections as designated after the midterm evaluation. Results of the election went as follow:
- Scientific Coordinators, Spokespersons
- Bianca Keilhauer (KIT) and Josef Jochum (Uni Tübingen)
- Administrative Coordinator and Deputy
- Andreas Haungs (KIT) and Marie-Christine Kauffmann (KIT)
- Coordinator Topic 1 - Astroparticle Theory and Deputy
- Günter Sigl (Uni Hamburg) and Karl Mannheim (Uni Würzburg)
- Coordinator Topic 2 - Non-Thermal Universe and Deputy
- Johannes Knapp (DESY) and Daniel Kuempel (RWTH Aachen)
- Coordinator Topic 3 - Dark Universe and Deputy
- Klaus Eitel (KIT) and Gernot Maier (DESY)
- Coordinator Topic 4 - Advanced Technologies and Deputy
- Matthias Kleifges (KIT) and Sebastian Böser (Uni Mainz)
- Coordinator Astronomy and Astrophysics and Deputy
- Martin Pohl (Uni Potsdam) and Markus Ackermann (DESY)
- Coordinator Astronomy and Astrophysics and Deputy
- Martin Pohl (Uni Potsdam) and Markus Ackermann (DESY)
- Coordinator LHC Physics and Deputy
- Thomas Lohse (HU Berlin) and Lutz Köpke (Uni Mainz)
- Coordinator Equal Opportunities
- Gisela Anton (Uni Erlangen) and Julian Rautenberg (Uni Wuppertal)
- Chair of the Member Board
- Christian Stegmann (DESY)
The 3 representatives of the Member Board to be in the Executive Board (EB) will be elected shortly. The EB will also now welcome an ex-Officio KAT representative. All details of the new member board can be found here and the revised HAP bylaws can be found on the HAP Sharepoint.
Two new Senior Fellows were also elected: Claus Grupen (Uni Siegen) and Hinrich Meyer (Uni Wuppertal). HAP also welcomed two new members with their groups: Thomas Bretz (RWTH Aachen) and Stefan Funk (FAU
Erlangen-Nürnberg).
Furthermore, it was announced that two new Associated
Partners joined HAP: the Gravitation AstroParticle Physics Amsterdam (GRAPPA) and
the National Institute for Subatomic Physics (NIKHEF), both from Amsterdam, Netherlands.
22.01.2015 - Gammateleskop H.E.S.S. entdeckt drei der leuchtstärksten Gammaquellen in der Nachbargalaxie der Milchstraße
Das H.E.S.S.-Teleskop-Array in Namibia, inzwischen durch ein großes 28-Meter-Teleskop erweitert.
(Bild: © Christian Föhr/ H.E.S.S. Collaboration, 2012)
Das Gammastrahlen-Observatoriuma H.E.S.S. (High Energy Stereoscopic System) stellt erneut sein großes Entdeckerpotenzial unter Beweis: In der Großen Magellanschen Wolke wies es extrem leuchtstarke Höchstenergie-Gammastrahlenquellen nach. Es handelt sich um drei verschiedenartige Objekte, nämlich den Pulsarwindnebel des stärksten je beobachteten Pulsars, einen extrem intensiv strahlenden Supernova-Überrest und eine sogenannte Superschale – ein 270 Lichtjahre großes schalenförmiges Gebilde, aufgeblasen von mehreren Supernovae und Sternen. Damit gelang es zum ersten Mal, in einer fremden Galaxie gleich mehrere sternähnliche Gammastrahlenquellen bei höchsten Energien zu beobachten. Zugleich ist die Superschale der erste nachgewiesene Vertreter einer neuen Klasse von Höchstenergie-Gammastrahlenquellen. Ihre Ergebnisse präsentiert die internationale H.E.S.S. Collaboration in der aktuellen Ausgabe des Wissenschaftsjournals „Science".
„In 210 Stunden Messzeit haben wir mit den H.E.S.S.-Teleskopen drei grundverschiedene Quellen hochenergetischer Gammastrahlung auflösen können“, sagt H.E.S.S.-Sprecher Prof. Christian Stegmann (DESY). „Das zeigt, was für ein riesiges Entdeckungspotenzial in der Nutzung kombinierter Systeme von Spiegelteleskopen steckt."
Kosmische Teilchenbeschleuniger, vor allem Supernova-Überreste und Pulsarwindnebel, also Endprodukte massereicher Sterne, sind Quellen sehr intensiver, höchstenergetischer Gammastrahlung. Sie beschleunigen geladene Teilchen auf extreme Geschwindigkeiten. Treffen diese auf das sie umgebende Gas oder Licht, entsteht die Gammastrahlung. Höchstenergetische Gammastrahlen aus dem Kosmos können mit hochempfindlichen Messgeräten auf dem Erdboden beobachtet werden. Beim Eintritt in die Atmosphäre verursachen sie Kaskaden geladener Sekundärteilchen, sogenannte Teilchenschauer. Diese emittieren extrem kurze, bläuliche Lichtblitze (Cherenkov-Licht), die mit großen Spiegelteleskopen und schnellen Lichtsensoren nachgewiesen werden.
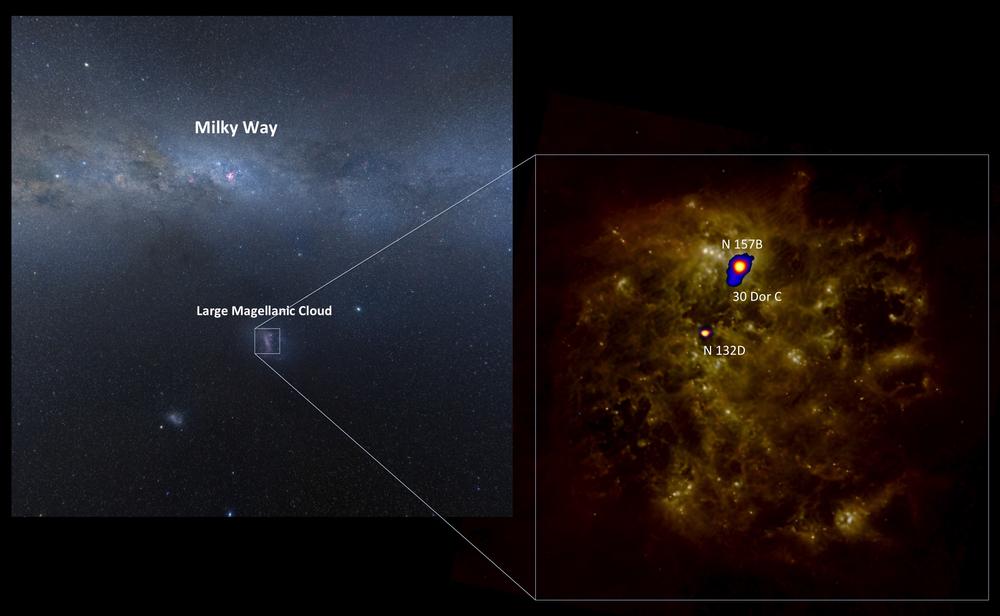
Optisches Bild der Milchstraße und eine Vergrößerung der Großen Magellanschen Wolke
im Infraroten mit darübergelegten H.E.S.S.-Himmelskarten.
(Milchstraße: © H.E.S.S. Collaboration, optisch: SkyView, A. Mellinger; LMC: © H.E.S.S. Collaboration,
infrarot: M. Braun et al. (1997), http://dirty.as.arizona.edu/~kgordon/research/mc/mc.html)
Die Große Magellansche Wolke (engl: Large Magellanic Cloud, LMC) ist eine Zwerg-Satellitengalaxie unserer Milchstraße in einer Entfernung von ungefähr 170 000 Lichtjahren. In ihr entstehen ständig neue Sterne, und sie beherbergt zahlreiche massereiche Sternhaufen. Die Rate, mit der neue massive Sterne gebildet werden und am Ende ihres Lebens als Supernovae explodieren, ist in der LMC im Verhältnis zu ihrer Masse fünf Mal höher als in der Milchstraße. Nicht zuletzt deshalb beobachten die Wissenschaftler der H.E.S.S. Collaboration dieses kosmische Objekt ausgiebig. Sie untersuchen dessen höchstenergetische Gammastrahlung, um den Mechanismus der Teilchenbeschleunigung in jungen Sternexplosionen genau zu verstehen.
Insgesamt 210 Stunden haben die Astroteilchenphysiker die H.E.S.S.-Teleskope auf die größte Sternentstehungsregion in der LMC gerichtet, den Tarantelnebel. Dabei gelang es ihnen zum ersten Mal, gleichzeitig mehrere Quellen höchstenergetischer Gammastrahlung in einer Galaxie außerhalb der Milchstraße räumlich aufgelöst abzubilden; dazu noch drei verschiedenartige, extrem energiereiche Objekte.
Bei der sogenannten Superschale „30 Dor C" handelt es sich um die größte bekannte Röntgenstrahlung emittierende Schale, die wahrscheinlich durch mehrere Supernova-Explosionen und starke Sternenwinde entstanden ist. Bisher war unklar, ob Superschalen zusätzlich zu einzelnen Supernova-Überresten kosmische Strahlung produzieren. Die Ergebnisse von H.E.S.S. zeigen, dass diese Superschale eine Quelle hochenergetischer Teilchen ist, mit denen sie gefüllt ist. 30 Dor C ist damit der erste nachgewiesene Vertreter einer neuen Klasse von Höchstenergie-Gammastrahlenquellen.
Der von den H.E.S.S.-Teleskopen beobachtete Pulsarwindnebel trägt die Nummer N 157B. Pulsare sind hoch magnetisierte, schnell rotierende Neutronensterne, die einen Wind ultrarelativistischer Teilchen emittieren und so einen Nebel bilden. Das bekannteste Exemplar ist der Krebsnebel, eine der hellsten Quellen am Hochenergie-Gammahimmel. Der Pulsar des Nebels N 157B ist in vielerlei Hinsicht ein Zwilling des sehr starken Krebspulsars in unserer eigenen Galaxis. Allerdings leuchtet N 157B im höchstenergetischen Gammalicht rund zehnmal heller als der Krebsnebel. Verantwortlich dafür sind das schwächere Magnetfeld in N 157B und das intensive Sternenlicht aus benachbarten Sternentstehungsgebieten, die beide die Erzeugung hochenergetischer Gammastrahlung fördern.
Der Supernova-Überrest N 132D ist bereits als helles Objekt im Radiowellen- und Infrarotbereich bekannt. Die jüngsten H.E.S.S.-Messungen zeigen, dass dieses Objekt außerdem einer der ältesten – und stärksten – Supernova-Überreste zu sein scheint, der noch im höchstenergetischen Gammalicht leuchtet. Er ist zwischen 2500 und 6000 Jahre alt und immer noch heller als die stärksten Supernova-Überreste in der Milchstraße, obwohl Modelle vorhersagen, dass in diesem Alter die Expansion der Supernovaschale schon so langsam sein sollte, dass sie kein effizienter Teilchenbeschleuniger mehr ist. „Die Ergebnisse bestätigen die Vermutung aus bisherigen Beobachtungen mit H.E.S.S., dass Supernova-Überreste wesentlich leuchtstärker sein können als bisher angenommen“, sagt DESY-Forscher Stefan Ohm, der maßgeblich an der Interpretation und Modellierung der LMC-Daten mitgearbeitet hat. Für ihre Messungen derartig dicht benachbarter Objekte hatten die Wissenschaftler insbesondere die Methoden zur Genauigkeit der Auflösung der Richtung, aus der die Gammastrahlung kommt, deutlich verbessert. Dies wird auch geplanten zukünftigen Experimenten der Astroteilchenphysik zugute kommen. „Stellen Sie sich nur vor, was wir mit dem viel empfindlicheren Cherenkov Telescope Array (CTA) in ein paar Jahren sehen werden!”, schwärmt Ohm.
Eine Elektronik-Modernisierung, die derzeit bei DESY in Zeuthen vorbereitet wird, wird ab 2016 die Leistungsfähigkeit des H.E.S.S.-Teleskopsystems weiter verbessern. Für die weitere Zukunft planen die Wissenschaftler das Cherenkov Telescope Array (CTA), das ab 2020 noch empfindlichere und höher aufgelöste Gammalicht-Bilder der LMC liefern soll. Auf der Wunschliste der Wissenschaftler für CTA steht diese Galaxie als wichtiges Beobachtungsobjekt weit oben.
H.E.S.S. - Das High Energy Stereoscopic System
In der H.E.S.S. Collaboration arbeiten Wissenschaftler aus Deutschland, Frankreich, Großbritannien, Namibia, Südafrika, Irland, Armenien, Polen, Australien, Österreich, Holland und Schweden zusammen, die von ihren jeweiligen Ländern und Institutionen unterstützt werden.
Die H.E.S.S.-Spiegelteleskope stehen in Namibia, im Südwesten Afrikas. Das System aus vier Teleskopen mit 13 Metern Durchmesser, das kürzlich mit dem riesigen 28-Meter-H.E.S.S. II-Teleskop ergänzt wurde, ist eines des empfindlichsten Nachweissysteme für höchstenergetische Gammastrahlen. Bei deren Eintritt in die Erdatmosphäre entstehen kurzlebige Teilchenschauer. Die H.E.S.S.-Teleskope registrieren die schwachen bläulichen Blitze, die die Teilchenschauer aussenden (genannt Cherenkov-Licht, einige Nanosekunden kurz), indem sie das Licht mit ihren großen Spiegeln sammeln und es auf die extrem empfindlichen Kameras reflektieren. Jedes Bild zeigt die Himmelsposition eines einzelnen Gammaphotons, und die gesammelte Lichtmenge entspricht seiner Energie. Photon für Photon kann H.E.S.S. so Karten der astronomischen Objekte im Gammalicht erstellen.
Die H.E.S.S.-Teleskope sind seit Ende 2002 in Betrieb; zum 10-jährigen Bestehen im September 2012 hatten die Teleskope in 9415 Beobachtungsstunden 6361 Millionen Luftschauer gemessen. H.E.S.S. hat die Mehrheit der etwa 150 bekannten kosmischen Objekte, die höchstenergetische Gammastrahlen emittieren, entdeckt. 2006 erhielt das H.E.S.S.-Team den Descartes-Preis der Europäischen Kommission und 2010 den Rossi-Preis der American Astronomical Society. 2009 reihte eine Studie H.E.S.S. in die Liste der zehn wichtigsten Observatorien weltweit ein.
|
Pressemitteilung: DESY
Publikation: The exceptionally powerful TeV gamma-ray emitters in the Large Magellanic Cloud, The H.E.S.S. Collaboration, Science, 2015, 347, issue 6220, p. 406 (arXiv:1501.06578)
16.01.15 - New Chair and General Secretary for APPEC
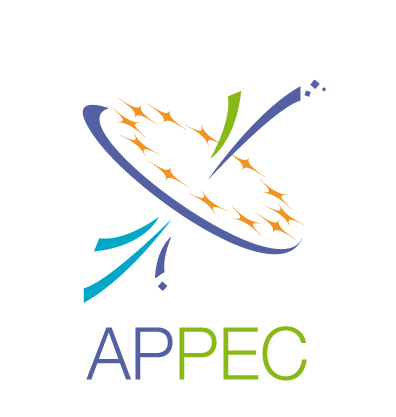
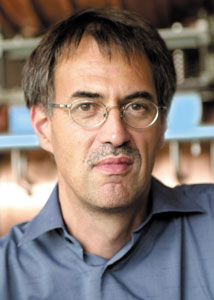
On January 9, 2015 the General Assembly of the Astroparticle Physics European Consortium (APPEC) elected Frank Linde – director of Nikhef from 2004 to 2014 – as its new Chair. He is taking over from Stavros Katsanevas, director of APC, who was chairing APPEC since November 2012. Thomas Berghöfer (DESY) was reappointed as General Secretary. Both appointments will be effective for the coming two years.
About his new appointment Frank Linde said: "Astroparticle physics addresses incredibly tantalizing and fundamental scientific questions. A top priority for me will be to get the large observatories in particular for multi-messenger studies really on track i.e. funded with a realistic spending profile taking into account exploitation costs. From APPEC's perspective we would also benefit from a stronger CERN involvement by learning from CERN’s very professional project review mechanisms as well as by hopefully attracting some of CERN’s resources to astroparticle physics e.g. for R&D activities".
Frank Linde also thanked Stavros Katsanevas for his dedicated work in leading the APPEC consortium and his enthusiastic
impact in forming the astroparticle physics community in Europe during his mandate.
Stavros Katsanevas summarized the first years of the APPEC Consortium taking over from the EU funded
ASPERA ERANET as quite active and formative: "Highlights of our activity have been the effort to
coordinate the community in view of Horizon 2020, the roadmap update and our policy contributions to
the European Particle Physics Roadmap as well as the P5 process in the USA, the preparation of the
triggering events and accompanying measures of the globalisation process in the neutrino sector and the
development of closer relationships with the astrophysics and particle physics communities (ERANET
ASTRONET, ECFA) and the international centres (ESO, CERN). I personally had great pleasure to
collaborate during these last years with the heads of agencies forming APPEC, Janet Seed (STFC) the
Vice-Chair of APPEC, Thomas Berghöfer the General Secretary, Antonio Masiero (INFN) the head of the
APPEC Scientific Advisory Committee, and of course the members of the APPEC functional centres.”
APPEC
Since its launch in June 2012, the APPEC consortium coordinates towards the next generation of
research infrastructures defined in its roadmap, including high energy multi-messenger observatories,
gravitational wave interferometers, experiments to derive neutrino properties and direct dark matter
searches as well as dark energy surveys.
The consortium currently comprises funding agencies from 14 European countries and ESO as an
observer. Negotiations are going on to enlarge the consortium by
partners from Armenia, Austria, Bulgaria, Czech Republic, Estonia, Finland and Portugal.
In the first half of 2015 APPEC is organizing a second global neutrino conference at Fermilab, a
Technology Forum on low-level light detection in Munich, and a cosmology workshop in Florence.
|
Press release: APPEC, Nikhef